Computation and experiment reveal how protein switching provides right tool for the job
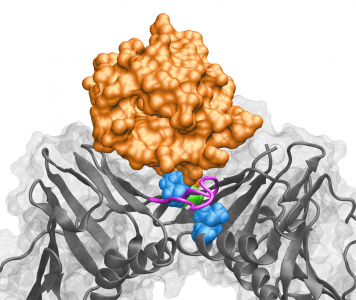
A model of ubiquitinated PCNA, refined using multiscale dynamics simulations and protein-docking experiments, shows ubiquitin (orange) binding in a groove above a PCNA subunit interface. Mutations known to interfere with translesion synthesis (blue and green) are directly beneath. The conformation of the J-loop (purple), a structural element of PCNA, is affected by mutations at the subunit interface. Image courtesy Ivaylo Ivanov.
Ultraviolet light, oxidants, and environmental toxins constantly assault the genome of a cell. Consequences in the absence of DNA repair could be severe—accelerated aging, degenerative disease, or cancer. Fortunately, cells have evolved sophisticated mechanisms for dealing with DNA damage, stabilizing their genomes, and ensuring their survival. Recently, a multi-institutional research team led by Ivaylo Ivanov of Georgia State University has employed the Jaguar XT4 supercomputer at Oak Ridge National Laboratory (ORNL) and x-rays a billion times brighter than the sun, produced at Lawrence Berkeley National Laboratory (LBNL), to illuminate how DNA replication continues past a damaged site so a lesion can be repaired later. The combination of computation and experiment reveals conformations that ubiquitin (Ub), a small protein that binds and orients DNA-editing enzymes, can assume when it associates with a molecular “tool belt” called proliferating cell nuclear antigen (PCNA). The results appear in the October 17, 2011, online issue of Proceedings of the National Academy of Sciences.
“The tool belt model is a longstanding model in the PCNA field, although it has not been conclusively proven,” said Ivanov, whose collaborators include Susan Tsutakawa of LBNL; John Tainer of LBNL and the Scripps Research Institute; Adam Van Wynsberghe of Hamilton College; Bret Freudenthal, M. Todd Washington, and Lokesh Gakhar of the University of Iowa College of Medicine; and Christopher Weinacht and Zhihao Zhuang of the University of Delaware.
“There are three binding sites on PCNA, which is a ring made of three identical subunits,” Ivanov explained. “The model proposes that each site can bind a different DNA-editing enzyme.” One such enzyme is DNA polymerase, which catalyzes the formation of a new DNA strand from an existing template. It also plays a major role in repair by associating with the tool belt and iteratively adding one of four bases to a damaged strand.
For the tool-belt model to be feasible, replicative and translesion polymerases need distinct binding sites. “Multiple polymerases—say, a high-fidelity replicase and a low-fidelity lesion bypass polymerase—could bind PCNA without steric clashes, forming a complex,” Ivanov said. “The model envisions that depending on the DNA context encountered by PCNA as it moves along DNA, it would swap the two polymerases and use the right tool from this set.”
Through the Innovative and Novel Computational Impact on Theory and Experiment (INCITE) program, the researchers received 2.6 million processor hours in 2009 on the Department of Energy Office of Science’s Jaguar XT4 to conduct their simulations. They also used the Intel 64 cluster Abe at the University of Illinois at Urbana-Champaign and its National Center for Supercomputing Applications through the National Science Foundation’s Teragrid.
The researchers used computing to winnow the number of places where Ub binds to PCNA from thousands of prospects to three. “Each stage of the refinement involved a different method with different timescale,” Ivanov explained. An application called Tethered Brownian Dynamics (TBD) explored the complex on the longest accessible timescale. It treats Ub and PCNA as rigid bodies as it quickly explores possible orientations in terms of electrostatic and geometric complementarity. With the electrostatic potential precomputed on a grid, interactions are approximate. RosettaDock, in contrast, uses a more sophisticated energy function, making it more accurate than TBD, and allows for side chain flexibility. It investigates protein–protein docking but keeps the backbone of both Ub and PCNA fixed to limit the expense of the conformational search. At the last stage, the researchers used the NAMD code to model the molecular dynamics of the entire system, including the solvent, in full flexibility. Clustering analysis bridged different stages in the multiscale modeling protocol.
“The job of the simulations is to provide low-energy, structurally compatible models that can then be vetted using the experimental data,” Ivanov said. Using LBNL’s Advanced Light Source (ALS), experimentalists obtained structures of Ub-PCNA complexes in solution using a technique called small-angle X-ray scattering (SAXS).
Combining multiscale computational modeling and SAXS showed that Ub didn’t have to reside just at the site on PCNA identified by the crystal structure; it could exist at two other sites that were computationally derived. The researchers called the positions “flexible,” “back,” or “side.” While the latter two indicate positions relative to PCNA, the flexible position is generated by high-temperature molecular dynamics, so the Ub becomes somewhat removed from the PCNA surface.
When PCNA traveling down a double strand of DNA bases encounters a stalled replication fork—a place where replication has stopped due to DNA damage—its first response is to attach a Ub molecule. Enzymes called translesion polymerases then attach to PCNA via Ub and replicate bases through the DNA lesion. That process is called translesion synthesis (TLS).
Finding new docking sites on the PCNA and equilibrium among the three Ub positions provides unexpected insight into previously unexplained biological observations. “The computationally identified positions explain the influence of mutations identified previously in genetic screens,” Ivanov said. “These are known to interfere with translesion synthesis but not with normal DNA replication.”
Like a construction worker swaps a hammer with a screwdriver on a tool belt to make a needed instrument more accessible, Ub can swap enzymes bound to PCNA when different ones are needed for TLS. “The dynamic range of positions offers an explanation of how the TLS polymerase could initially bind to the back side of PCNA and then transition to the polymerase binding face of PCNA,” Ivanov said.
Simulations rule out a hypothesis
Normally, replicative DNA polymerases carry out DNA synthesis. These enzymatic proteins are exquisitely tuned to incorporate the correct nucleotide. This efficiency and accuracy also limits what types of DNA lesions the enzymes can accommodate in their active sites. If a lesion cannot be accommodated, replication stalls, threatening cell survival. If replication is not allowed to proceed, apoptosis, the pathway that programs cells for death, is activated.
“Fortunately, other pathways can take over that do not result in cell death,” Ivanov said. “Translesion synthesis is one of the principal pathways for dealing with the situation of stalled replication forks.”
After TLS begins with the attachment of Ub to PCNA, the Ub-PCNA complex recruits TLS polymerase, which is specially adapted to deal with DNA damage. This class of polymerase can progress past the DNA lesion like a train, with the flip of a switch, can bypass a bad section of railroad track.
How the TLS polymerase displaces the regular polymerase remains unclear, but simulations ruled out one hypothesis. “Allosteric models have been suggested where Ub modification induces changes in the structure of PCNA and that conformational change, in turn, displaces the replicative polymerase. From both the PCNA-Ub structure and from the simulation, it is clear that this is not the case,” Ivanov said. “Another option for achieving the polymerase switch is for Ub to increase the binding affinity of TLS polymerase to its substrate and in effect out-compete the replicative polymerase. We support this latter option, since the Ub is flexibly attached to PCNA and, by transitioning from the crystallographic to the computationally identified position, may assist the movement of the TLS polymerase from the back to the front face of PCNA to displace the replicative polymerase.”
The researchers received an INCITE allocation of 4 million processor hours in 2010 and 2011, which they ran on ORNL’s Jaguar XT5 supercomputer. Next they aim to develop and apply novel multiscale computational protocols, which would allow integrative modeling of complex biological assemblies. Results from this line of research, specifically for DNA replication and repair complexes, could lead to biomedical advances in the diagnosis, prognosis, and treatment of cancer and inherited disorders.
Georgia State University, the Cleon C. Arrington Research Initiation Grant Program, the National Cancer Institute, the National Institute of General Medical Sciences, and the National Science Foundation supported the work.—by Dawn Levy
Related publications:
Tsutakawa et al. 2011. “Solution X-ray Scattering Combined with Computational Modeling Reveals Multiple Conformations of Covalently-Bound Ubiquitin on PCNA.” Proceedings of the National Academy of Sciences, 108 (43): 17672-17677. doi: 10.1073/pnas.1110480108.