Ounce for ounce, carbon fiber could quickly become the wonder material of the future. The material, already used in a variety of products, boasts densities comparable to plastic, strengths comparable to steel and versatilities comparable to rubber under the right conditions. Its fibers, spun from organic carbon polymer strands thinner than a human hair, can take the place of metal in auto and aircraft parts, lighten the load in running shoes and even shore up skyscrapers.
Bringing that future to the present depends on scientists finding cheaper and easier ways to mass-produce carbon fiber, which costs more to make on average than its rival materials. A study by researchers at the U.S. Department of Energy’s Oak Ridge National Laboratory used the Summit supercomputer, along with modeling and simulation techniques applied in the nuclear nonproliferation field, to help bring the carbon-fiber revolution closer to reality. The study takes a first step toward building a scalable computer model to examine the material’s properties, predict its strengths and weaknesses, and offer suggestions on how to streamline its production.
Findings appeared in Physical Chemistry Chemical Physics.
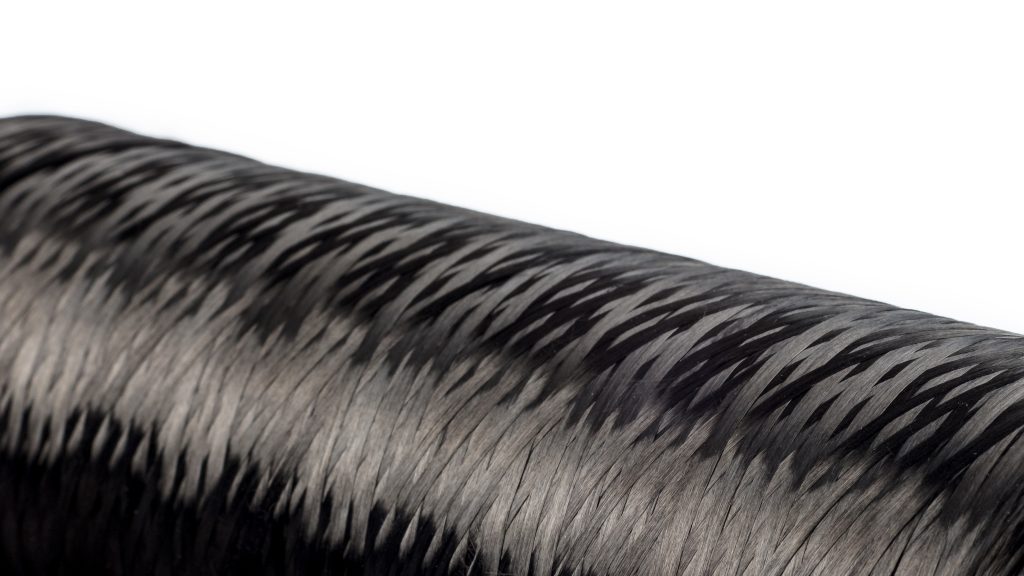
A spool of carbon fiber.
Credit: Carlos Jones/ORNL
“Carbon fiber is everywhere now, but it’s still costly to fabricate, and the structural details are surprisingly complex,” said Jennifer Niedziela, an ORNL physicist and co-author of the study with ORNL’s Sara Isbill, Ashley Shields and Roger Kapsimalis. “If we can model carbon fiber virtually and translate that modeling to process-monitoring capability, then we can potentially make major improvements in the production process with less time and expense.”
To make those improvements, researchers need greater insight into carbon fiber’s individual polymers at the atomic level.
“We have to understand how these polymers interact and how each of those interactions contributes to the material’s overall behavior,” Niedziela said. “Then we can understand the material as a whole and translate those lessons into practical applications.”
Carbon fiber’s superpower comes from its high strength-to-weight ratio. In other words, a little carbon fiber can hold up and hold together a lot of mass, but it’s still not clear whether defects can act as a fiber’s kryptonite. The research team sought to identify at the atomic level which defects play what roles in that ratio and how to tweak potential combinations to boost carbon fiber’s strength and mitigate weaknesses.
“We need to better understand the atomic-level structure,” Isbill said. “Eventually, we want to look at these materials under conditions outside their normal equilibrium, such as higher temperature, and see how they respond. This study is a first step toward that.”
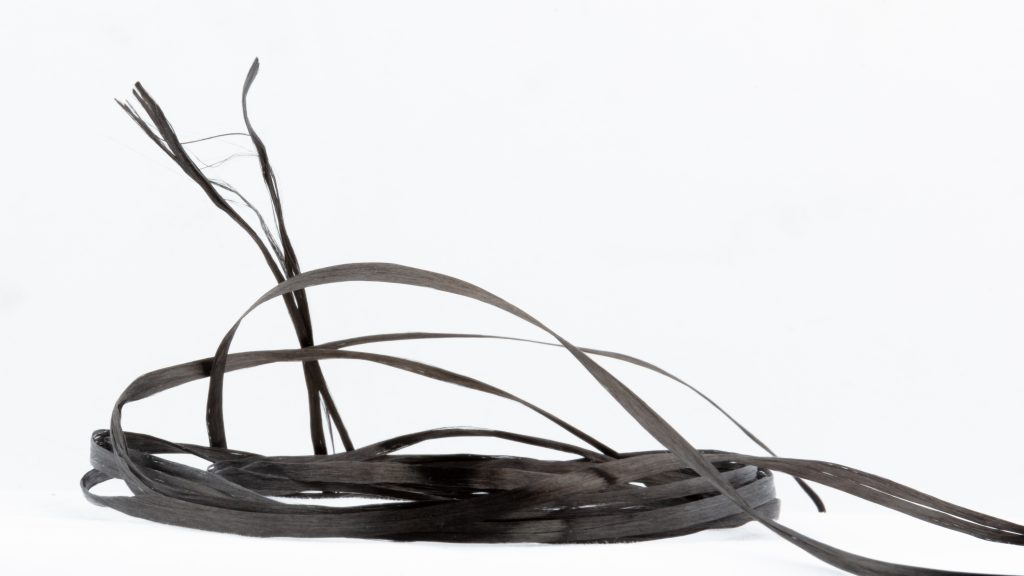
Strands of carbon fiber.
Credit: Carlos Jones/ORNL
Researchers typically use Raman spectroscopy to bombard samples of carbon fiber with photons and measure the light’s interaction with the carbon fiber’s chemical bonds. The difference in energy lost or gained by the photons interacting with the material offers a kind of fingerprint for the sample measured and reveals how the atoms vibrate within the structure. Peaks in the Raman spectra relate to the atomic structure, but sometimes other experiments or computational models may be needed to clarify those relationships.
For this study, the team used density functional theory, a quantum-mechanical approach to estimating materials’ electronic and atomic structures, to calculate the structure and vibrational properties of two separate defects in a 400-atom carbon fiber cell.
The complicated calculations used to simulate these cells – defects and all – required the computational power of Summit, the Oak Ridge Leadership Computing Facility’s 200-petaflop IBM AC922 supercomputing system.
“As soon as we start incorporating defects, it breaks up the symmetry of our model and requires more calculations to simulate, which means we can’t use the usual approximations and other shortcuts to lower computational costs,” Isbill said. “We couldn’t have done this without Summit.”
The simulations focused on the Dienes defect, a flaw that can occur in bonds between carbon atoms, and monovacancy, or removal of an atom (carbon in this case) from the structure. Findings yielded new insights into the structural changes caused by both defects.
“Thinking of carbon fibers as highly defective graphite, these calculations on Summit move us another step closer to being able to simulate a realistic, complex carbon-fiber system at the atomic level and accurately predicting key material properties,” Shields said.
The study won’t lead to construction of an all-carbon-fiber skyscraper tomorrow. But the findings offer another step toward a model that could help maximize carbon fiber’s potential by making it stronger, cheaper and more useful to industry.
“Carbon fibers offer so many possibilities for such a wide range of applications that there’s broad and growing interest in this field and plenty of opportunities for big-scale science,” Isbill said. “We’re looking forward to exploring this further.”
Support for this research came from the DOE Office of Science’s Advanced Scientific Computing Research Leadership Computing Challenge. The OLCF is a DOE Office of Science user facility.
Related publication: Sara Isbill et al., “Computational Investigations of Dienes Defect- and Vacancy-Induced Changes in the Electronic and Vibrational Properties of Carbon Fiber Structural Units,” Physical Chemistry Chemical Physics 23 (2021): 27385–27396, https://doi.org/10.1039/D1CP03930J.
UT-Battelle LLC manages Oak Ridge National Laboratory for DOE’s Office of Science, the single largest supporter of basic research in the physical sciences in the United States. DOE’s Office of Science is working to address some of the most pressing challenges of our time. For more information, visit https://energy.gov/science.