With the help of supercomputers, scientists are getting a better idea of how to study some of the strongest electromagnetic fields in the universe.
Scientists aim to use high-power lasers to blast different materials and study the interplay between light and matter. Quantum electrodynamics (QED) is the study of how super small particles that make up these two interact with one another. A team led by Henri Vincenti, a research scientist at the Commissariat à l’Energie Atomique (CEA) in France, hopes to reach the strong-field QED regime, which is characterized by particles of light being emitted from, or decaying into, charged particles. Using lasers or collisions of heavy nuclei in particle accelerators, scientists can attempt to reach this regime, but even some of the most powerful lasers on Earth aren’t sufficient to reach it. The fields in this regime are so extreme that extraordinary physical processes can take place within them.
Vincenti’s team recently used the fastest supercomputer in the United States, Summit, which is housed at the US Department of Energy’s (DOE’s) Oak Ridge National Laboratory (ORNL), to model a new technique designed to boost lasers to much higher intensities and help scientists pin down the strong-field QED regime. The results were published in Physical Review Letters.
“The processes occurring in strong-field QED happen in some of the most extreme astrophysical scenarios you can think of,” said Luca Fedeli, postdoctoral researcher at CEA. “Phenomena such as black holes, gamma ray bursts, or the magnetospheres surrounding a neutron star are very difficult to study on Earth. So, when we say ‘strong field,’ we really mean that. This electric field is huge.”
So huge that if the field were to be attained in a pure vacuum, it would rip the vacuum apart. Nuclear physicists recently reported observing this phenomenon in a particle collider, as atomic nuclei passed by each other at near the speed of light. The results took years to confirm and relied on a sophisticated analysis to separate other possible effects. A setup with lasers may allow similar field strengths to be achieved in a more stable arrangement.
Vincenti proposed using an optical device called a relativistic plasma mirror, which could take the most intense lasers available today and render them 1,000 times more powerful. The team modeled Vincenti’s idea on Summit and found that the scenario would likely trigger strong-field QED processes, in which particles of light, or photons, decay into positively charged positron particles and negatively charged electron particles.
“Our simulations suggest it should be possible to significantly boost the yield of positrons generated when the laser interacts with the target, indicating that we would then be in this strong-field regime,” Fedeli said.
A doppler-boosted laser beam (blue) interacting with a secondary target (grayscale). The laser hits the secondary target, and the high-energy photons (orange) decay into electrons and positrons (green and violet) when they cross very strong-field regions. Video Credit: Luca Fedeli, Commissariat à l’Energie Atomique
The results could aid scientists studying astrophysical scenarios such as black holes and neutron star mergers, thereby offering new perspectives on phenomena that have been difficult to understand with current experimental methods.
Boosted lasers pack a bigger punch
Vincenti’s main goal is to study what happens when an electric field is so strong that it generates matter, a phenomenon called the Schwinger effect. To generate this field in the laboratory, ultra-intense lasers are needed.
The current record for ultra-intense lasers on Earth is the equivalent of all the solar radiation currently striking the surface of Australia focused down to an area the size of a bacterium. However, the team needs lasers with a million times greater intensity than even that extreme example to reach the Schwinger field.
Vincenti proposed boosting current ultra-intense lasers by using plasma mirrors, which are typically solid targets like slabs of glass that are irradiated by the laser and then turn into plasma almost immediately. Using the Oak Ridge Leadership Computing Facility’s (OLCF’s) Summit under an Innovative and Novel Computational Impact on Theory and Experiment (INCITE) allocation, the team modeled a laser hitting a plasma mirror and then reflecting off the plasma onto a secondary target. The OLCF is a DOE Office of Science user facility at ORNL.
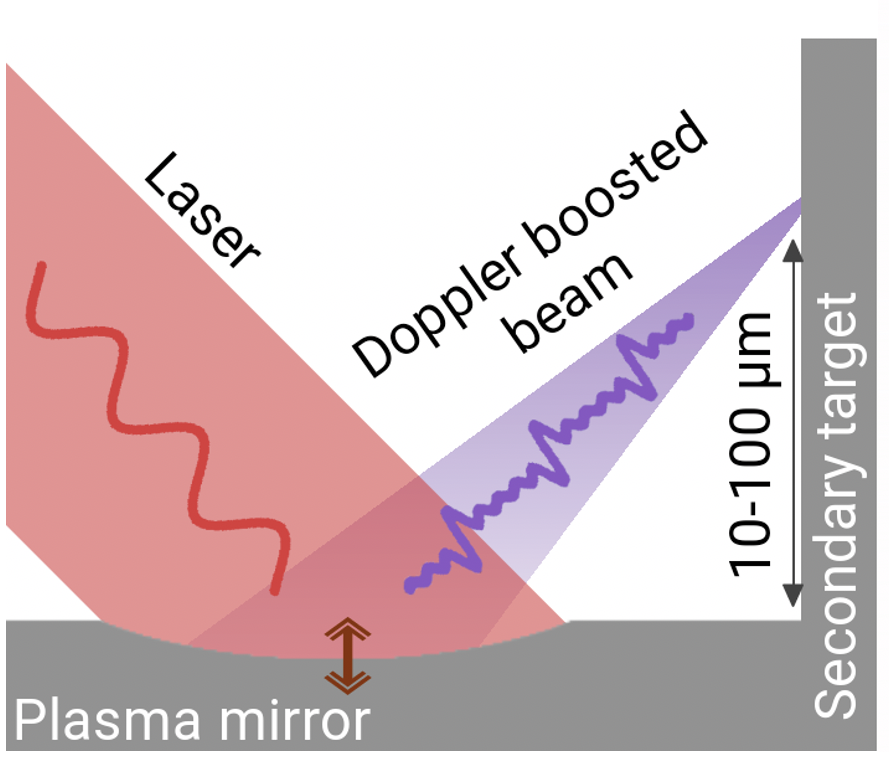
In the proposed scheme for probing SF-QED with present-day or near-future lasers, a plasma mirror shaped by radiation pressure converts an intense laser pulse (red) into Doppler-boosted harmonics (purple) and focuses them on a secondary target, reaching extreme intensities. The dimensions involved are tens to hundreds of microns (millionths of a meter); the diameter of a human hair is a few to several tens of microns. Credit: Luca Fedeli, CEA
“We predict orders of magnitude greater yield from a scenario where we just focus a laser as much as possible onto a target to a situation where we use the scheme we developed with this secondary target,” Fedeli said.
The team used an L-shaped target to perform a detailed investigation of the interaction region using a particle-in-cell (PIC) code called WarpX, an Exascale Computing Project code that Jean‑Luc Vay, senior physicist at Lawrence Berkeley National Laboratory, is developing with his team. PIC codes use an electromagnetic field modeled on a grid and employ macro particles to represent generic plasma particles. These two components interact, the macro particles generating currents that are then used as an electromagnetic source for the grids.
The team’s PIC code is unique because it uses a pseudospectral solver, which can decompose signals, including laser signals, into basic functions. This allows the team to employ fast Fourier transforms, which break down electromagnetic signals into their various spectral components and thereby enable an accurate prediction of their evolution for all frequencies.
“This feature is essential for the simulations to be able verify the generation of strong-field QED effects with the proposed scheme with great fidelity,” Vay said.
Summit enables the study of a stronger regime
The team needed Summit’s capabilities to explore the characteristics required to identify whether they were in the strong-field QED regime. Each of the team’s 33 simulations required several dozen or even hundreds of compute nodes on massive grid sizes.
“Summit allowed us to explore a wide range of parameters, to run many simulations with different parameters and see what happens,” Fedeli said.
The team’s simulations suggest that, with existing or soon-to-be-existing technology, they will reach quantum parameters that will enable them to explore strong-field QED experimentally. The team plans to simulate the same scenario in 3D in the future.
“In the surroundings of objects like black holes and neutron stars, you have conditions suitable for strong-field quantum electrodynamics processes to take place,” Fedeli said. “Being able to explore such processes in a laboratory using lasers, as proposed in our scheme, will give us further confidence in our models.”
This work also received support from DOE’s Exascale Computing Project, the French National Research Agency (ANR) T-ERC program, the Cross-Disciplinary program on Numerical Simulation of CEA, French Alternative Energies, the Atomic Energy Commission, and the European Union’s Horizon 2020 research and innovation program.
Related Publication: Luca Fedeli, Antonin Sainte-Marie, Neil Zaïm, Maxence Thévenet, Jean-Luc Vay, Andrew Myers, Fabien Quéré, and Henri Vincenti, “Probing Strong-Field QED with Doppler-Boosted PetaWatt-Class Lasers,” Physical Review Letters 127 (2021): 114801, doi:10.1103/PhysRevLett.127.114801.
The research was supported by DOE’s Office of Science. UT-Battelle LLC manages Oak Ridge National Laboratory for DOE’s Office of Science, the single largest supporter of basic research in the physical sciences in the United States. DOE’s Office of Science is working to address some of the most pressing challenges of our time. For more information, visit https://energy.gov/science.