Using the Summit supercomputer at the US Department of Energy’s (DOE’s) Oak Ridge National Laboratory (ORNL), a team at the Arizona State University Biodesign Institute has confirmed details of a molecular mechanism that cell organelles use to regulate the pH of their environment—and it looks a lot like a Ferris wheel.
Led by Abhishek Singharoy, a researcher at the Biodesign Center for Applied Structural Discovery (CASD) and Arizona State University’s School of Molecular Sciences (SMS), the team used Summit to simulate molecular pumps that are embedded in the fatty outer membranes of cellular organelles, or cell structures that are specialized to perform different tasks for the cell’s functioning. With rotors that spin 100 times per second, these pumps rapidly move protons—positively charged subatomic particles—into a cell’s organelles to maintain the acidic environment inside that is crucial to the work they perform.
Using the nation’s fastest supercomputer—the Oak Ridge Leadership Computing Facility’s (OLCF’s) Summit—the team uncovered a key step in how this mechanism works in one proton pump in particular, a yeast proton pump called vacuolar ATPase (V-ATPase) that is necessary for a variety of tasks in the body and has implications in disease. The results were published in Science Advances.
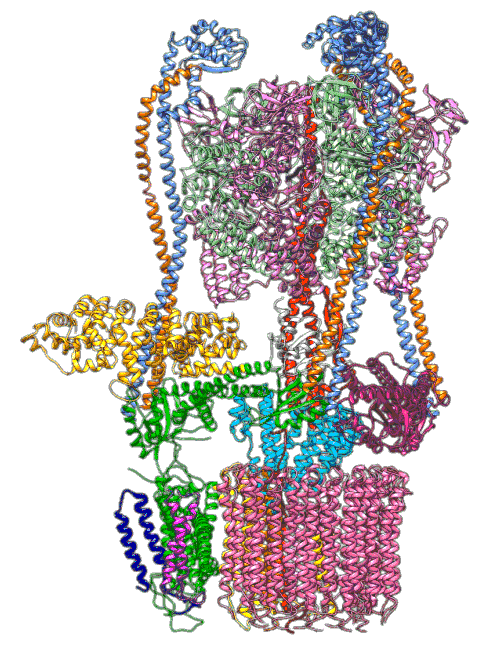
An animation shows a proton pump called V-ATPase at work. These pumps are embedded in the membranes of cellular organelles, where they bring in protons that are essential for the organelle’s function. The top part of the pump generates energy to drive the rotating part at the bottom, which is like a molecular Ferris wheel that picks up protons on the outside of the organelle and drops them off inside. Scientists used cryo-EM images and computer simulations to reveal key details about how the pump works. Image Credit: S.-H. Roh and S. Wilkens
“The V-ATPase proton pumps perform a wide range of functions, from helping transmit nerve signals to helping specialized cells secrete acid for maintaining bone,” said Stephan Wilkens, a biochemist at SUNY Upstate Medical University and study co-author. “Malfunctions in these molecular machines contribute to diseases such as osteoporosis, neurodegeneration, diabetes, cancer, and AIDS, so understanding them is important for human health.”
A team led by Wah Chiu—professor at the SLAC National Accelerator Facility and Stanford University and codirector of the Stanford-SLAC Cryo-EM Facilities—together with Wilkens and SLAC/Stanford postdoctoral researcher Soung-Hun Roh, had already performed work at SLAC that employed Cryogenic electron microscopy (cryo-EM) to study these proton pumps. Images from the work showed 10 amino acid “seats” on a molecular Ferris wheel that bind protons and carry them through the membrane to the organelle’s interior, as well as other amino acids that catch them when they arrive. Based on that picture, the team working on the research suggested that the proton drop-off might be aided by water molecules—but their images were not sharp enough to confirm that the water molecules were there. In the same study, another round of even higher resolution cryo-EM images showed the water molecules around the suspected proton path.
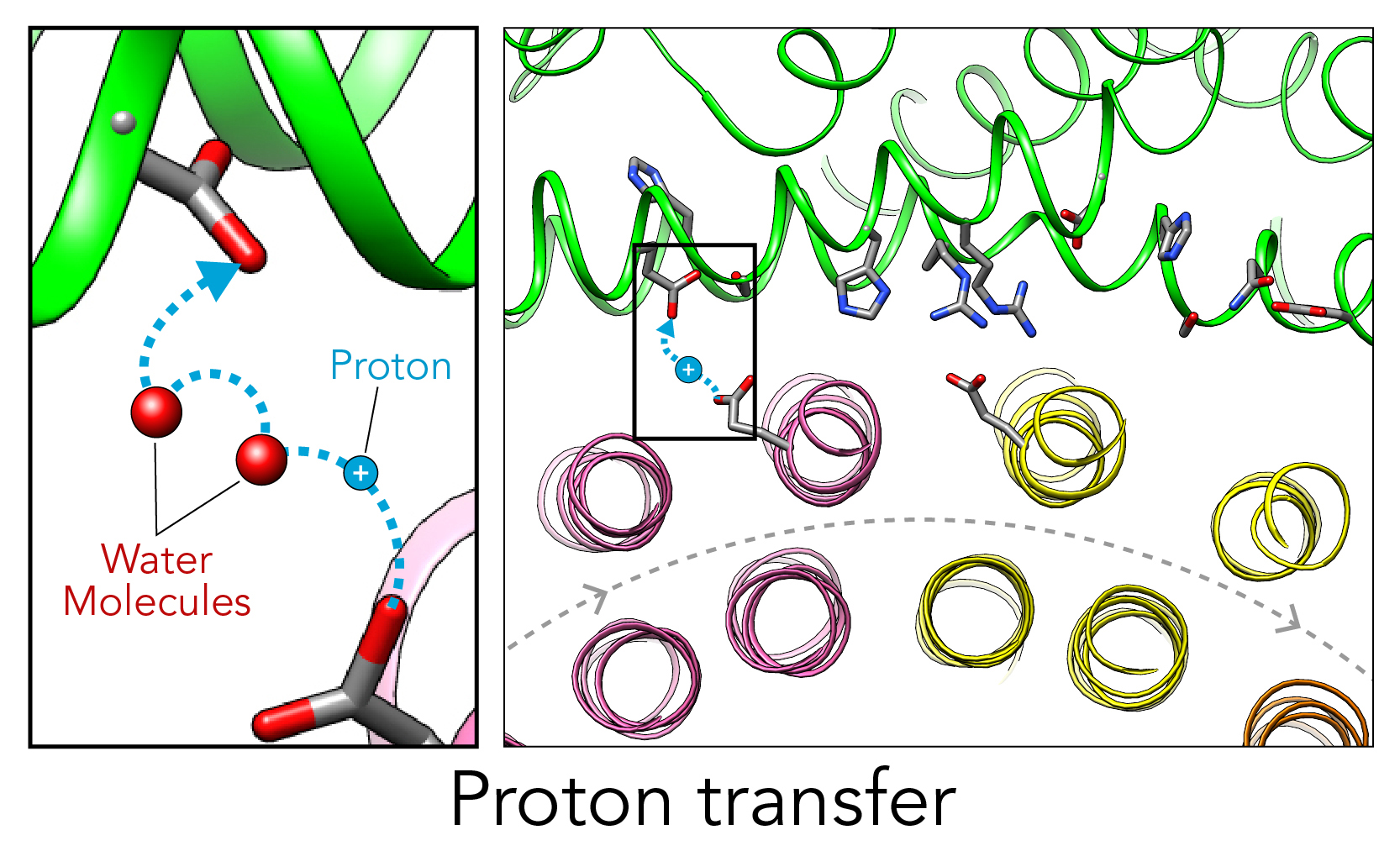
In this illustration, the Ferris wheel part of the V-ATPase proton pump is at the bottom, rotating clockwise, and the inside of the organelle—the drop-off point for protons—is at the top, in green. The zoomed-in view at left depicts a key finding of this study: Scientists confirmed that water molecules form “wires” at the drop-off point that convey protons from their seats on the Ferris wheel to the organelle interior, like a fire brigade passing buckets hand to hand. Image Credit: Greg Stewart/SLAC; S.-H. Roh et al., Science Advances, 7 October 2020
Singharoy and his team used the Nanoscale Molecular Dynamics, or NAMD, code to perform simulations on the Summit supercomputer and confirmed that the experimentally observed water molecules line up to form “wires” at the proton drop-off point. These wires convey protons from their seats on the Ferris wheel to landing spots inside the organelle, like a fire brigade passing buckets hand to hand, bridging a gap they couldn’t navigate on their own. The work was performed under the Innovative and Novel Computational Impact on Theory and Experiment, or INCITE, program at the OLCF.
The simulations matched the cryo-EM images nicely, providing strong evidence that the picture they painted of the proton drop-off is correct.
“Molecular motors exemplify some of the most intricate chemo-mechanical devices, and our team in SMS and CASD has developed highly sophisticated computational tools to address the energy source and sinks of the motor’s ratcheting motion,” Singharoy said. “In 2017 we started working on the soluble part of the V-type motor, namely, V1 ATPase. Now that we have a good control on the transmembrane Vo motor, it’s a great step forward toward simulating the entire motor in collaboration with Hun, Stephan, and Wah.”
Chiu said that recent advances in cryo-EM that allow imaging of individual particles at atomic resolution—even when they take slightly different shapes—will open new opportunities for using it as a tool to discover effective drugs for illnesses involving proton pumps.
The OLCF is a DOE Office of Science User Facility located at ORNL.
Related Publication: Soung-Hun Roh, Mrinal Shekhar, Grigore Pintilie, Christophe Chipot, Stephan Wilkens, Abhishek Singharoy, and Wah Chiu, “Cryo-EM and MD Infer Water-Mediated Proton Transport and Autoinhibition Mechanisms of Vo Complex,” Science Advances 6, no. 41 (2020): eabb9605. doi:10.1126/sciadv.abb9605.
This story was written by Glennda Chui at SLAC and adapted by Rachel McDowell at the OLCF.
UT-Battelle LLC manages Oak Ridge National Laboratory for DOE’s Office of Science, the single largest supporter of basic research in the physical sciences in the United States. DOE’s Office of Science is working to address some of the most pressing challenges of our time. For more information, visit https://energy.gov/science.