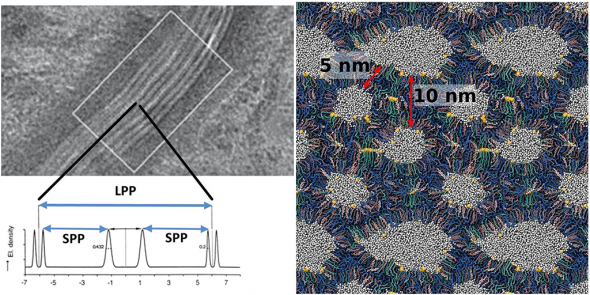
Microscopy (left) shows layers of the stratum corneum’s waxy “mortar” in which keratinaceous “bricks” called corneocytes are embedded. The layers contain repeated short- and long-chain lipids. Molecular dynamics simulations performed on Titan (right) demonstrate that the long-chain fatty acids are crucial to keeping the skin barrier intact. Image credits: left, modified from Iwai et al., Journal of Investigative Dermatology 132, 2215-2225 (2012) and Bouwstra et al., Journal of Lipid Research 39, 186–196 (1998); right: Michael Klein, Giacomo Fiorin, and Christopher MacDermaid (Temple University) and Russell DeVane (Procter & Gamble)
Oak Ridge National Laboratory simulation predicts epidermal responses to compounds
Skin is the body’s largest organ. It is a protective barrier, keeping microbes out and moisture in. It also regulates temperature, enables sensation, and makes vitamin D. But researchers don’t fully understand at the molecular level how our skin performs its functions.
Companies developing products applied to skin, such as lotions, body washes, and cosmetics, need a deep understanding of how ingredients molecularly interact with this barrier. They need to know which chemical compounds are blocked by the skin, which are absorbed, and which compromise its structural integrity. Moisturizers are meant to permeate skin, for instance; cosmetics are formulated mainly for the surface.
So in 2013, researchers at consumer-products giant Procter & Gamble (P&G) and Temple University began using the Titan supercomputer at the Department of Energy’s (DOE’s) Oak Ridge National Laboratory (ORNL), to better understand the three-dimensional structure of skin’s outermost barrier, the stratum corneum. This barrier is composed of dead cells that are impermeable to some compounds and embedded in lipids like bricks set in mortar. No quantitative model existed that could predict with certainty the amount of a specific molecule that could cross the skin.
With Titan, the second-most-powerful computer in the world, the researchers achieved in a matter of weeks three milestones that would have taken years with their in-house high-performance computers. First, they simulated a 1-million-atom section of the stratum corneum’s lipid mortar that revealed how skin can be simultaneously permeable and resilient when exposed to agents that transform it. Second, they used their molecular dynamics simulation to measure the quantity, rate, and pathway of chemicals permeating lipids of the stratum corneum to validate high-throughput models. Third, they gained important new insights into the mechanisms by which some compounds disrupt skin.
“The multilamellar, multicomponent structure of skin is one of the most complicated lipid systems one can study,” said Temple’s Michael L. Klein, a National Academy of Sciences member who led the project and has collaborated with P&G for 15 years. Russell DeVane of P&G and Giacomo Fiorin of Temple were his project partners.
“Skin lipids are complex mixtures in 3D arrangements that no one fully understands,” Klein said. “Through modeling and simulation we aim to improve our molecular-level understanding of skin penetration.”
Improved understanding of skin permeation is important to P&G, an $85 billion firm established in 1837 that started off in soap. (Ivory was among its first brands.) Today its product lines encompass beauty and grooming (e.g., CoverGirl and Crest), health and wellbeing (e.g., Pepto-Bismol and Vicks), and household care (e.g., Dawn and Tide). It has 25 brands with net sales exceeding $1 billion, including Olay, Pantene, Wella, and Head & Shoulders. “It’s hard to think of a product that we have that doesn’t come into contact with your skin at some point,” said P&G’s DeVane.
The company has long relied on research to innovate, but the process of testing its products is expensive and time-consuming, using skin from human cadavers, surgical patients, and pigs, as well as synthetic skin. Replacing these physical tests with computational tests that model human skin could significantly reduce costs and accelerate screening of ingredients, bringing new and improved products to market faster.
No-sweat screening
“We wanted to probe molecular-level interactions between compounds and the skin in order to understand how to build more detail into our models so we can do more accurate high-throughput computational screening,” said DeVane. The screens would accurately predict how a compound permeates full skin.
Screening simulations use a model that Professor Jerry Kasting at the University of Cincinnati developed with experimental data. Two key inputs to the model are a compound’s molecular weight and its octanol–water partition coefficient (a ratio of concentrations of the compound after it has settled into two immiscible phases). Both parameters are available in scientific literature.
“When you want to screen a large number of compounds through the Kasting model, the beauty is you don’t have to do experimental measurements beforehand to characterize the compounds,” said DeVane, who aims to screen thousands of compounds in mere minutes.
To help achieve this goal, he and his colleagues are using the Kasting model as a base to build a fuller model of skin by adding a more detailed representation of the stratum corneum lipid matrix, through which compounds must pass to enter skin. They are refining this more complex model by using large-scale molecular dynamics simulations to show with greater realism how chemicals permeate skin. Future simulations may allow them to accurately predict how specific compounds interact with skin lipids.
While existing models like Kasting’s correctly predict a compound’s skin permeation most of the time, according to DeVane, instances of incorrect predictions can happen for reasons not fully understood. Unreliable predictions typically underestimate permeation.
“If you want to use models to ensure zero risk [of unwanted skin permeation] and make some statement about exactly what makes its way through the skin, you don’t want to underestimate permeation,” DeVane warned.
“These large-scale molecular dynamics simulation are our best option for filling in the gaps in our understanding of skin permeation and explaining the deviations we observe in the current model. Those new insights will be built into an augmented Kasting model to improve the accuracy of its predictions,” he added.
Super model meets supercomputer
P&G has owned several generations of supercomputers. Today the firm operates a 300-core hybrid cluster with traditional central processing units (CPUs) and accelerated graphics processing units (GPUs) in addition to larger CPU-only resources with more than 10,000 cores. Temple’s capabilities are similarly impressive and include an 864-core cluster that also has eight GPU-equipped nodes. But the challenge at hand surpassed the capabilities of even those high-performance machines.
So the researchers turned to ORNL’s industrial partnerships program, which provides companies with high-impact science or engineering projects access to the Oak Ridge Leadership Computing Facility (OLCF), a DOE Office of Science user facility. This access helps companies accelerate research likely to improve their success in competitive markets. The industrial partnerships program provides access through three means: the Innovative and Novel Computational Impact on Theory and Experiment (INCITE) program, which supports large-scale projects; the Office of Advanced Scientific Computing Research Leadership Computing Challenge, which supports projects of special interest to DOE; and the Director’s Discretion program, which focuses on national needs.
Through INCITE, in 2013 Klein’s team ran codes for 86.8 million core hours on the OLCF’s flagship supercomputer. Computational scientist Arnold Tharrington, the project’s OLCF liaison, ensured the collaborators made the most of Titan during several months of molecular dynamics simulations that each day mirrored the movements of skin molecules over 60 to 70 nanoseconds. The simulations also covered responses to ingredients applied to skin. The supercomputer divided the simulations into pieces for faster processing.
“Every node of Titan is GPU-enabled,” DeVane said. “That acceleration was critical to meeting our needs.” The INCITE allocation, which exploited Titan’s 18,688 accelerated nodes, allowed the researchers to gain insights in 1 month that would have taken 8 years on Temple’s 864-core cluster, Fiorin added.
To model the stratum corneum for the project, the researchers used the LAMMPS and NAMD molecular dynamics codes. With NAMD able to simulate biological systems in full atomistic detail and LAMMPS renowned for coarse-grained simulations of large systems, each code is well suited to make use of DOE’s large supercomputers.
At ORNL the researchers predominantly used the GPU-accelerated NAMD code to track the movements of every molecule in the stratum corneum lipid model to see how it evolved over time. NAMD calculates a thermodynamically equilibrated arrangement—the most energetically “comfortable” configuration for atoms in a lipid molecule to assume.
Covering the findings
Access to Titan enabled three major achievements. The first was simulation of 1 million atoms of skin lipid matrix—four separate bilayers, each hundreds of square nanometers and made of ceramides (waxy compounds), fatty acids, and cholesterol in water. This skin simulation, extending over 2 microseconds, was far too large and complex to have been carried out without OLCF resources. After 600 nanoseconds at the temperature at which ceramides start to melt, the bilayers morphed into hexagons that segregated water inside. These watery pockets contribute to the formation of pores and may be one of the means by which hydrophilic (water-loving) ingredients penetrate skin. When restoring conditions to body temperature, the ceramides and other lipid molecules recovered order and cohesion, but the watery pockets remained. This phenomenon showed that ceramide melting is one way of making skin permeable while keeping it whole.
The second major achievement enabled by the INCITE allocation was providing validation to components of the Kasting model. In particular, the researchers used large-scale simulations to model the permeation of compounds through the stratum corneum, showing that assumptions in the Kasting model were valid for a broad range of compounds.
The researchers’ third major accomplishment was modeling the contact between skin and large concentrations of selected chemical compounds to uncover the mechanism by which compounds disrupt the stratum corneum barrier. Skin’s highly ordered lipids imbue its barrier property, and ceramide plays a key role. The mechanism showed how compounds could essentially melt this waxy substance, but chemically instead of thermally.
In addition to those achievements, the researchers are trying to get a handle on the molecular structure of skin. Lipid composition plays an important part in the stratum corneum’s structure and consequently the skin’s barrier properties. Experimentalists have shown that changing the composition alters the structure and, thereby, the barrier properties of the skin.
“We know the complex mixture of lipids is important for the skin barrier properties, but we don’t know the role each lipid component plays,” DeVane said. “Our simulations are attempting to shed light on why nature picked this specific lipid composition.”
Radiant future
Before the project, skin had only been visualized microscopically or with simpler simulation models. Now because of this project, the researchers have investigated its structure in full atomistic detail. This new knowledge is a good foundation for molecularly probing what factors hold skin together and which ones threaten its stability.
To scratch the surface of those mysteries, Klein, DeVane, and Fiorin have received a 2014 INCITE allocation of 75 million core hours for computations using a combination of LAMMPS and NAMD.
Meanwhile, most of the information from the 2013 INCITE project will be published to benefit a global community eager to develop computational methods to replace costly, slow, inefficient experiments. Through the European Chemical Industry Council, P&G is working on a skin-penetration model with rival Unilever, chemical company BASF, the University of Warwick, and the University of Cincinnati. The benefits are likely to extend beyond the consumer-products industry. For example, a better understanding of skin permeation could enable the pharmaceutical industry to advance drugs that are administered through the skin.
P&G, along with Jerry Kasting and researchers from the University of Illinois at Chicago and the University at Buffalo, the State University of New York, have also received a National Science Foundation grant to study skin-modeling techniques. “We’re looking at different modeling techniques besides molecular simulations to help us understand and get at longer timescale issues. The INCITE project’s more realistic model will boost the success of these efforts,” DeVane said.
The global skin-care business was valued at nearly $100 billion in 2012, according to Euromonitor International, and the global beauty market may reach $265 billion in 2017, according to Lucintel, a market research firm.
“If a better understanding of skin brings improvements that allow us to capture even 5 percent more of a market, that’s a huge return on investment,” DeVane said. “That’s why P&G has skin in the game.”
Related Publications and Presentations
Marketa Paloncyova, Russell H. DeVane, Bruce P. Murch, Karel Berka, and Michal Otyepka, “Rationalization of Reduced Penetration of Drugs Through Ceramide Gel Phase Membrane,” Langmuir (2014). https://dx.doi.org/10.1021/la503289v
Talk delivered at the University of Pennsylvania April 1, 2013, downloadable as “MacDermaid_Fiorin_IRG” from https://www.copy.com/s/AOGe4syQ0Ud1/20140401_IRG2
Poster presentation delivered at a Temple University bioengineering symposium April 30, 2013, downloadable from
https://www.dropbox.com/s/ojyzjr3qvl2uyvb/TIME-Symposium-low-qualty.jpg