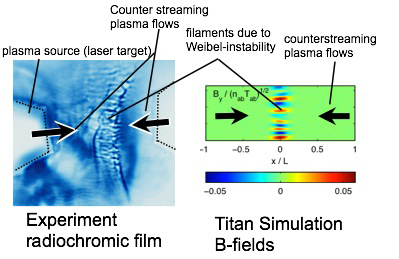
The collisionless shock physics was probed through a concerted effort of both experiments on the OMEGA EP laser facility and first-principles plasma simulations
Conducted on Titan through INCITE.
Researchers use Titan to unravel the many mysteries of high-density plasmas
One of the greatest assets of simulation is its ability to help researchers refine experiments.
In some cases simulation is never meant to provide the ultimate answer; rather, it enables researchers to design more effective experiments that will do exactly that, only much more efficiently than without the aid of simulation.
Such is the case with one group’s study of magnetic reconnection, the process by which magnetic field lines in a highly ionized gas, known as a plasma, break and reconnect, releasing a substantial amount of energy. Reconnection is studied around the world (and in outer space) in numerous disciplines, and simulation is an increasingly vital tool in its investigation.
“Magnetic reconnection is a fundamental plasma process,” said Amitava Bhattacharjee, a professor in the Department of Astrophysical Sciences at Princeton University and head of the Theory Department of the Princeton Plasma Physics Laboratory (PPPL). Wherever there are opposing magnetic fields interacting, there is reconnection, from solar radiation interacting with Earth’s magnetosphere to fusion reactions for clean energy.
Of particular importance to Bhattacharjee’s team, which includes leading experimentalists Will Fox of PPPL and Gennady Fiksel of the University of Rochester’s Laboratory for Laser Energetics, is reconnection and shocks in systems where the plasma particles do not collide very often, both of which can serve as mechanisms for cosmic ray acceleration.
Although reconnection can be studied in outer space via satellites, it is also studied here on Earth in laboratories. Due to its nature, however, studying reconnection in a laboratory setting can be challenging and expensive, hence the need for simulation.
And for simulation, few tools are better than Titan, the Oak Ridge Leadership Computing Facility’s Cray XK7 supercomputer, currently America’s fastest for open science. In Bhattacharjee’s case, Titan helped his team design experiments on one of America’s most powerful lasers, Omega.
Housed at the University of Rochester’s Laboratory for Laser Energetics, Omega is a fundamental tool for studying the nature of plasmas in general, from those in the far reaches of the universe to those created in hopes of generating electricity via a fusion reaction.
And because one shot on Omega can equal thousands of dollars, the importance of refining is difficult to overstate. “Experiments always bring out new things,” said Bhattacharjee, adding that the “success of the experiments relied on preparation, in which simulation played a vital role.”
And success in this case meant not one, but two major breakthroughs in the complex field of magnetic reconnection.
The importance of bubbles
The PPPL-Rochester team was initially motivated by pioneering experiments carried out by groups at MIT and in the United Kingdom. Those researchers appeared to have found reconnection rates that were apparently “extraordinarily large” compared with theoretical predictions.
“It was the paradox that drove us,” said Bhattacharjee. “These simulations helped resolve the discrepancy and provided invaluable insights on new experiments that went well beyond the scope of earlier experiments. For example, it was found that prior to the main experiment, it would be important to fill the volume with a low-density plasma (called a pre-plasma), which would enable better coupling of the two main driver plasmas with the background magnetic field. This was critical in our success with the experiments at Rochester.”
The simulations go as follows: plasmas are struck with powerful lasers, such as Omega, which create bubbles containing magnetic fields that break and reconnect. Such reconnection processes are thought to play a large part in the solar atmosphere and in the magnetospheres of stars, planets, and black holes—areas where places where two magnetized plasmas meet.
“These magnetic fields act like rubber strings,” said Bhattacharjee. “They are unwound, and that energy is released in the form of heat and particles . . . If you can make sense of experiments quantitatively with these codes, then maybe we have some reliable basis for extrapolation to astrophysical objects.”
In the case of reconnection, the team’s simulations validated what had been observed in experiments, solidifying our knowledge of its role. In fact, these simulations were refined to Omega’s plasma configuration, validating the results and confirming previous experiments and guiding new ones.
Yet another breakthrough
But that wasn’t all. Another set of experiments revealed the theoretical Weibel instability, a phenomenon in plasmas with ion beams streaming in opposite directions. The finding was detailed in the November 27, 2013, issue of Physical Review Letters.
In fact, this was the first laboratory observation of this important astrophysical phenomenon, first theorized in 1959.
The instability is important for two reasons, namely for providing an improved fundamental understanding of plasma physics and for its role in cosmic shock waves that are critical to astrophysical explosions and the creation of magnetic fields during the formation of galaxies.
The Weibel simulations were nearly identical to the team’s reconnection experiments, except that in the Weibel case no magnetic field was applied.
Using particle-in-cell codes, in which individual particles are tracked over time, the PPPL-Rochester team followed the motions of ions and electrons, solving the entire set self-consistently, or together.
“These simulations have all the detailed physics that should matter,” Bhattacharjee said. “While such detailed simulations have limitations in terms of size, we do remarkably well in terms of realism.”
Observation of the Weibel instability was achievable through careful preparation and analysis, both of which were led by realistic simulations with Titan. Those simulations allowed matching of the most relevant dimensionless parameters for essentially one-to-one comparison with recent experiments, something far out of the reach of smaller systems.
Bhattacharjee and his collaborators acquired time on Titan through the Innovative and Novel Computational Impact on Theory and Experiment (INCITE) program. Open to researchers from academia, government labs, and industry, INCITE is the major means by which the scientific community gains access to some of the fastest supercomputers.
“INCITE gave us the computer time we needed to do simulations ahead of our experiments, enabling us to plan experiments so that they were successful,” said Bhattacharjee.
As Bhattacharjee’s team has shown, the realms of simulation and experiment are not exclusive. In fact, when used together, they are a single, unifying tool for expediting scientific breakthroughs.