Jaguar enables researchers to choose between contradictory experiments
The oxygen-23 isotope is rare and ephemeral.
This is not the oxygen that keeps your body running. It exists at the edge of the nuclear landscape, where its neighbors are always fleeting and most commonly found within exploding stars.
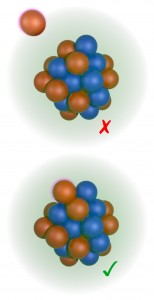
A halo neutron resides outside the nucleus. Oxygen-23 does not have such a nucleus.–Illustration by Andy Sproles, ORNL
Nevertheless, if we are to understand how the universe is put together, we must understand exotic isotopes such as oxygen-23. A research team from Oak Ridge National Laboratory (ORNL), the University of Tennessee (UT) and the University of Oslo in Norway recently contributed to this understanding with intense calculations of the oxygen-23 nucleus performed on ORNL’s Jaguar supercomputer. In doing so the researchers also demonstrated that supercomputer simulation has become an indispensable tool for scientific discovery, on par with physical experiment. Their work is discussed in a recent edition of the journal Physical Review C.
When we think of oxygen we’re usually thinking of oxygen-16, whose nucleus has eight positively charged protons and eight uncharged neutrons. This isotope makes up well over 99 percent of the naturally occurring oxygen, and it doesn’t decay. It is especially important to sustaining life, both as the stuff that keeps us breathing and as the heavier of water’s two elements. When you step on a scale, oxygen-16 is nearly two-thirds of the weight that stares back up at you.
With eight protons and 15 neutrons, oxygen-23 does decay—and quickly. The oxygen-23 nucleus has a half-life of 82 milliseconds, meaning that if you have 10,000 atoms now you’ll be down to two or three within a second. Its neighbor, oxygen-24, is believed to be the heaviest an oxygen isotope can get; beyond it lies the so-called neutron drip line, where neutrons will no longer attach to a nucleus. While they may be rare, these and other exotic isotopes are important, at least in part because they challenge current theories of how a nucleus—and therefore the universe—is constructed.
“Our goal is to explain the origin of the elements,” explained Gaute Hagen of ORNL, who did the calculations with Øyvind Jensen of the University of Oslo and Thomas Papenbrock of ORNL and UT. “The only way to go beyond oxygen on the nuclear chart is to go via paths that are along the very drip line of the nuclear chart. On a bigger scale, astrophysics is also nuclear physics. What happened in the first milliseconds after the Big Bang, that’s nuclear physics as well.”
Nuclear physicists have worked for more than 60 years within an approach known as the nuclear shell model, akin to the atomic shell model that governs electron orbits. The nuclear model notes that as you add protons or neutrons to a nucleus, it becomes especially stable at certain numbers such as 2, 8, and 20. These are known as magic numbers. Calculated for protons and neutrons separately, they indicate that a “shell” within the nucleus has been filled. In addition, there are “subshells” in between these numbers where the nucleus is relatively stable, but less so than with a full shell.
Magic isotopes
This model does well describing stable isotopes, but it becomes problematic as you move toward unstable, exotic nuclei. For instance, the model would suggest that oxygen-28, with eight protons and 20 neutrons, is magic, yet no such isotope has been observed and researchers believe oxygen-24 is the heaviest possible oxygen isotope. In fact, a recent paper from Hagen and colleagues in the journal Physical Review Letters supports the contention that oxygen-24 itself is magic, although existing theory would say otherwise.
By exploring exceptions to the shell model as it has been understood, researchers seek a deeper understanding of all nuclei, stable and unstable alike.
“The idea is that these naïve shell model pictures of the nucleus do not hold when you go to the very extreme of the nuclear chart,” Hagen noted, “where you have very neutron-rich or unstable or fragile systems.”
Enter oxygen-23. The oxygen nucleus reaches a relatively stable subshell with 14 neutrons at oxygen-22. The 23rd neutron is essentially left over. Experimental data from a decade ago suggested that the 23rd neutron did not even touch the others, but rather hovered over the nucleus as a halo. That conclusion grew from observation that the nucleus had an especially large cross section; in other words, it was very wide.
Data from more recent experiments by Rituparna Kanungo of Saint Mary’s University in Halifax, Nova Scotia, disagreed. Working at Germany’s GSI Helmholtz Centre for Heavy Ion Research, Kanungo concluded that the cross section of oxygen-23 was far smaller, meaning that it did not have a neutron halo.
The question, then: which experiment was right?
Kanungo invited Hagen to explore the question computationally, which he did with a first-principles approach known as coupled cluster theory. To ensure the most accurate possible answer, he did the calculations for several oxygen isotopes, from oxygen-21 with 13 neutrons to oxygen-24 with 16. Each calculation ran on about 100,000 processors and used several million processor-hours.
A tough calculation
Oxygen-23 is a very neutron-rich nucleus, which until very recently could not be accurately analyzed microscopically with existing supercomputers. Hagen noted that the calculations necessary to handle 23 strongly interacting particles were very complex and required a system of Jaguar’s power.
“This work could not have been done without Jaguar,” he said. “It would not be possible. It’s putting us at the forefront of our field just being at this location.”
The calculations showed that Kanungo’s conclusion was right and oxygen-23 indeed does not have a halo.
“It’s not a halo nucleus, our calculations verified,” Hagen said. “We computed the ground state, the radius of the system, and the density profile of the neutrons to see where the neutrons really are. By using those results and the reaction calculation, Kanungo was able within the uncertainty of the experiment to put our calculations in there, and there was very nice agreement between theory and experiment.”
The study of this isotope is a piece in the puzzle of nuclear physics. As supercomputers grow in power, they will tackle ever-heavier isotopes and, possibly, help us understand how matter is put together.
“It affects our understanding of the universe, its evolution, and why we’re here,” Hagen said. “All those questions relate to what we do, and they are really not located at the stable part of the nuclear chart. They’re at the extremes.”
Related publications
“Exploring the anomaly in the interaction cross section an matter radius of 23O,” R. Kanungo et al., Phys. Rev. C 84 061304 (2011), DOI: 10.1103/PhysRevC.84.061304.
“Continuum effects and three-nucleon forces in neutron-rich oxygen isotopes,” G. Hagen et al., Phys. Rev. Lett. 108 242501 (2012), DOI: 10.1103/PhysRevLett.108.242501.