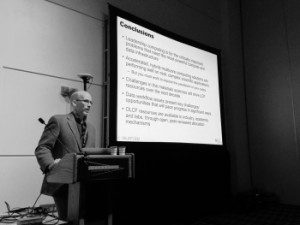
Jack Wells, OLCF Director of Science, chaired the first and third sessions on scientific computing of the March Meeting of the American Physical Society.
Thirty-Seven Titan Users Contribute Presentations
This year’s March Meeting of the American Physical Society featured a focus session on computational materials research proposed and led by Oak Ridge National Laboratory’s (ORNL) Jack Wells and Thomas Schulthess.
The March Meeting is the world’s largest gathering of physicists, with approximately 10,000 scientists attending annually. The session called “Petascale Science and Beyond: Applications and Opportunities for Materials,” comprised three 3 hour sessions, with 3 invited speakers, and 36 shorter contributed presentations. Wells, who serves as the Oak Ridge Leadership Computing Facility’s director of science, chaired the first and third sessions. Schulthess, who is director of the Swiss National Supercomputing Center and a professor at ETH Zurich as well as an ORNL staff researcher, chaired the second.
The three invited speakers were Prof. David Ceperley, a theoretical physicist at the University of Illinois at Urbana–Champaign; Prof. Joost VandeVondele, head of the nanoscale simulations group at ETH Zurich: and Prof. Aurel Bulgac, a physics professor at the University of Washington. Ceperley discussed applications of the quantum Monte Carlo method. The Monte Carlo method simulates systems through random sampling; quantum Monte Carlo is the application of this technique to quantum systems.
VandeVondele discussed recent progress in using density functional theory (DFT) on petascale supercomputers. DFT is a method of modeling quantum mechanical systems. It can determine a many-body system’s electronic structure and is thus useful in computational physics, chemistry, and materials science.
Bulgac’s presentation was on low-temperature physics simulations he had done on the OCLF’s Titan supercomputer. He suggested that a unique waveform recently observed in an ultracold material called “superfluid unitary Fermi gas” was in fact merely a tiny vortex ring, as supported by DFT simulation of the superfluid.
Of the 96 authors of contributed presentations, 37 were Titan users. They included ORNL’s Markus Eisenbach and Jan-Michael Carrillo. Eisenbach authored or coauthored three presentations, all focusing on magnetic systems research.
“One of the interesting questions in physics is really, what is the ground state of a system?” he explained. “That is, if it were at zero absolute temperature, what state would the system be in? Another question is, what if you have a finite temperature?”
When Eisenbach says “states,” he doesn’t mean solid, liquid, or gas. He’s talking about magnetic states. Magnetism occurs because the magnetic moments of atoms or molecules line up with one another. Magnetic states hinge on how magnetic moments are aligned. Temperature can affect magnetic state because heat randomizes a system; the hotter an atom is, the less likely it is that its magnetic moment will align with the magnetic moments around it.
Eisenbach’s work involves using basic physical laws to simulate the magnetic state of Ni2MnGa—a material containing nickel, manganese, and gallium—at different temperatures. He used two simulation paradigms: he either calculated every possible state of a small (48 atom) sample (called exact enumeration), or simulated a few randomly selected possible states of a larger sample (Monte Carlo sampling). Ni2MnGa is of interest because of a strong magneto-caloric effect; that is, it shows large temperature changes as its magnetic state changes. The purpose of this simulation was to figure out what exactly is happening at the molecular scale when this transition occurs.
At the March Meeting, Eisenbach and his coworkers discussed exact enumeration of Ni2MnGa’s magnetic states, Monte-Carlo sampling of Ni2MnGa’s states, and Wang-Landau sampling (the specific type of Monte-Carlo sampling that he used).
Carrillo’s work involves organic photovoltaic cells, a potentially lower-cost alternative to the silicon-based solar cells currently on the market. Most organic photovoltaics contain four components: an electron donor, an electron acceptor, and two electrodes. In the type that Carrillo is simulating, called bulk heterojunction photovoltaic cells, the donor material (in this case, the polymer P3HT) and the acceptor material (here fullerene derivative PCBM) are mixed together, instead of being separated as in traditional bilayer cells. This increases efficiency, since electricity is produced only at the interfaces between the two types of materials.
To produce a bulk heterojunction photovoltaic, P3HT and PCBM are heated to encourage phase-segregation (in which the electron donor and acceptor form separate homogenous domains within the material). To see what happens at the molecular level during this process, known as thermal annealing, Carrillo simulated it on Titan, using 21 percent of the machine’s full capacity.
Hoping to find the optimal combination of materials to use, Carrillo varied the length of the polymers being simulated, tested mixtures of long and short polymer chains, and tried different concentrations of each component.
“Experimentalists are often very influential people in [the physics community],” Wells said, “and if possible I don’t want them to miss the contributions that petascale science is making.”
The petascale science focus sessions helped spread the word regarding the possibilities supercomputing provides for physicists. —Timothy Metcalf