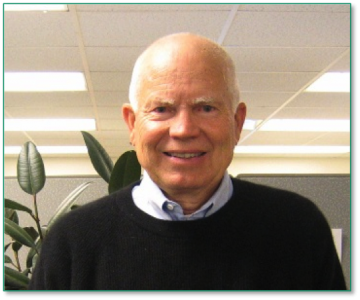
Doug Scalapino, researcher of physics at UC-Santa Barbara, has benefited from using both OLCF and SNS resources.
Neutron scattering and supercomputing power the search for a common thread
In this conversation with Douglas Scalapino, a research professor of physics at the University of California at Santa Barbara, he describes his work with ORNL’s premier user facilities. A leading theorist in condensed matter physics, Scalapino is a fellow of the American Physical Society and a member of the National Academy of Sciences. He is a recipient of the John Bardeen Prize for theoretical work in superconductivity and the Julius Lilienfeld Prize for outstanding contributions to physics, as well as numerous prestigious research fellowships.
Scalapino’s research has benefited from leading ORNL facilities–specifically the Spallation Neutron Source (SNS) and the Oak Ridge Leadership Computing Facility (OLCF). He was the inaugural speaker for a new joint lecture series sponsored by the SNS and ORNL’s Center for Nanophase Materials Sciences. His lecture, “Common thread: The pairing interaction for a class of unconventional superconductors,” addressed the question of whether there is a common link among known types of unconventional superconductors.
Superconductivity arises when electrons in a material form pairs that can flow without resistance through the atomic structure, or lattice, of the material. In conventional superconductivity, scientists accept that electron pairing results from an attractive interaction between the electrons, mediated by vibrations of the lattice (phonons) that overcome the natural repulsion between the negatively charged electrons.
However, in materials known as unconventional superconductors, the electron pairing is thought to be the result of some other mechanism. After decades of research, scientists still don’t agree on precisely what that mechanism is. In his talk, Scalapino described a spin-fluctuation pairing mechanism he believes is the common thread linking the known types of unconventional superconductors–copper oxides (cuprates), iron-based, heavy fermion, and organic. In the spin-fluctuation mechanism, an exchange interaction between electron spins (“spin” is angular momentum) is such that as an electron moves, it creates a wake in the local antiferromagnetic spin correlations, which attracts a second electron. This pairing interaction is therefore most likely to be found in materials that are nearly antiferromagnetic (having a regular pattern of spins pointing in opposite directions), which is the case for unconventional superconductors.
Q: Since the discovery of cuprate high-temperature superconductors in the 1980s, what findings have contributed the most to understanding unconventional superconductivity?
A: The discovery of the iron-based superconducting materials has played a significant role in our thinking. This discovery supported the idea that there was a class of unconventional superconductors and that it made sense to look for a common pairing mechanism. In 1986, there was a group of superconducting materials called heavy fermions, as well as some organic materials, and it was suggested that the superconductivity in these materials was mediated by spin fluctuations rather than phonons. In this case, fluctuations of the electron spins, rather than fluctuations of the ion lattice, provide the attractive pairing interaction. When the cuprates came along, some felt they were part of this same family. But then we theorists spent years working on the many fascinating properties of the cuprates and tended not to think so much about family relationships. Then the iron materials came along, and it appears that the iron materials are like the cuprates, and they’re part of this family of superconductors going by the same mechanism.
This is reassuring. We [theorists] haven’t spent 25 years trying to understand just the cuprates. You know, that’s the knock on the theorists–that we’ve spent 25 years wandering in the wilderness making up all these different theories. We did work on the cuprates for a long time. But the nice feature to me is that there appears to be a large class of materials that go by a similar mechanism–and that class includes heavy fermions, the plutonium actinide materials that were more recently discovered, the cuprates, the iron materials. So there’s a large class–the cuprates aren’t an isolated system. There’s a common feature.
Q: What are the most exciting things learned from the iron-based superconductors?
A: A friend of mine, when he heard about the high-temperature cuprate superconductors for the first time, remarked to me, “If I’d gone out into my back yard and seen a green Martian step off a spaceship, I would not have been more surprised.” Now with the discovery of the iron superconductors, we realize “we are not alone”–it’s not just the cuprates. To me, that is what is so striking: It brought home the fact that there may be lots of materials out there that hold the promise of higher-temperature superconductivity.
Q: Does the evidence suggest there are multiple paths to high-temperature superconductivity?
A: There seem to be multiple theories as to how to get to high-temperature superconductivity. However, looking at the unconventional superconductors we now have, I believe that what’s remarkable is that it appears they all have a common pairing mechanism.
Other pairing mechanisms have been proposed. For example, H. Kantana (Nagoya University, Japan) has a theory in which the electron pairing is mediated by orbital fluctuations. It’s a very interesting theory, and we may find that happening in some material. If we do, it will strongly couple with the lattice vibrations, the phonons, so it will be both orbital and phonon in nature. That would be exciting–it would be like conventional superconductivity on steroids. Another possible mechanism is charge fluctuations, which people have looked for since the early 1960s.
It’s not that I doubt there is the possibility of other mechanisms. But if I have to put money on it, I think in all the unconventional materials we’ve talked about, the superconductivity is driven by spin fluctuations, that is, by the same mechanism. I think that at present there appear to be two classes of superconducting materials–one driven by electron-phonon interactions and the other by spin fluctuations.
Q: How are high-performance computing and neutron scattering moving the field forward?
A: Neutron scattering is central in that it measures the spectrum of spin fluctuations, measures that these materials have a tendency for antiferromagnetism, and measures their phase diagrams. Finally, the observation of a peak in the neutron scattering that appears in the superconducting state provides evidence of the unconventional nature of the superconducting state. This peak arises from an interference effect associated with the structure of the pair wave function of the unconventional superconductors. For example, for the cuprates, it tells us that the electron pair has d-wave symmetry. For the iron-based materials it tells us that the pair has what is called s± structure. The neutrons have been crucial. They’ve played a central role both in looking for the pairing glue, the spin fluctuations, and in looking to see whether we’re dealing with an unconventional superconductor.
Calculations carried out at ORNL’s National Center for Computation Sciences (NCCS) have allowed us to determine the pairing interaction in models that have been used to describe these materials, such as Hubbard models. Here the important point is that numerical calculations have also shown that these models exhibit a number of the properties that are seen in the actual materials. So it is natural to ask what causes the pairing in these models, and our calculations have shown that it’s the spin fluctuations.
Q: What are the areas where understanding is converging, where scientists think they’re zeroing in on key aspects of unconventional superconductivity?
A: Do we have a theory of the high-temperature superconducting cuprates? Actually we have a number of theories, but there is not yet a consensus.
I think it’s converging experimentally to a belief that there are strong spin fluctuations in these materials. However, recent neutron scattering measurements suggest there are magnetic excitations in the cuprates that are different from the spin fluctuations, and of somewhat unknown origin. There’s not agreement on the explanation for this, and some believe these “hidden modes” drive the superconductivity. So there are pieces of this puzzle we don’t yet understand; however, I don’t think that this means that we can’t understand the pairing mechanism. After all, we’ve had 25 years and we’ve learned a lot about the cuprates, and I think some of the properties we don’t understand are peripheral to the mechanism.
The remarkable thing that’s happened in the last 25 years is the development of experimental techniques that give us atomic-level resolution and incredible frequency and momentum resolution. The measurements are beautiful and detailed, putting immense constraint on the theories, which is good–we need to be restrained. There are a lot of techniques and a lot of materials. These provide key insights. Then the question comes, when do we put it together? And the last question is, when do we agree we’ve put it together?
Interview by Deborah Counce, science writer for SNS